What is Reactive Oxygen Species? How they are harmful? And Their Mitigation Strategies?
- Tirmazi Syed
- Apr 25, 2021
- 8 min read
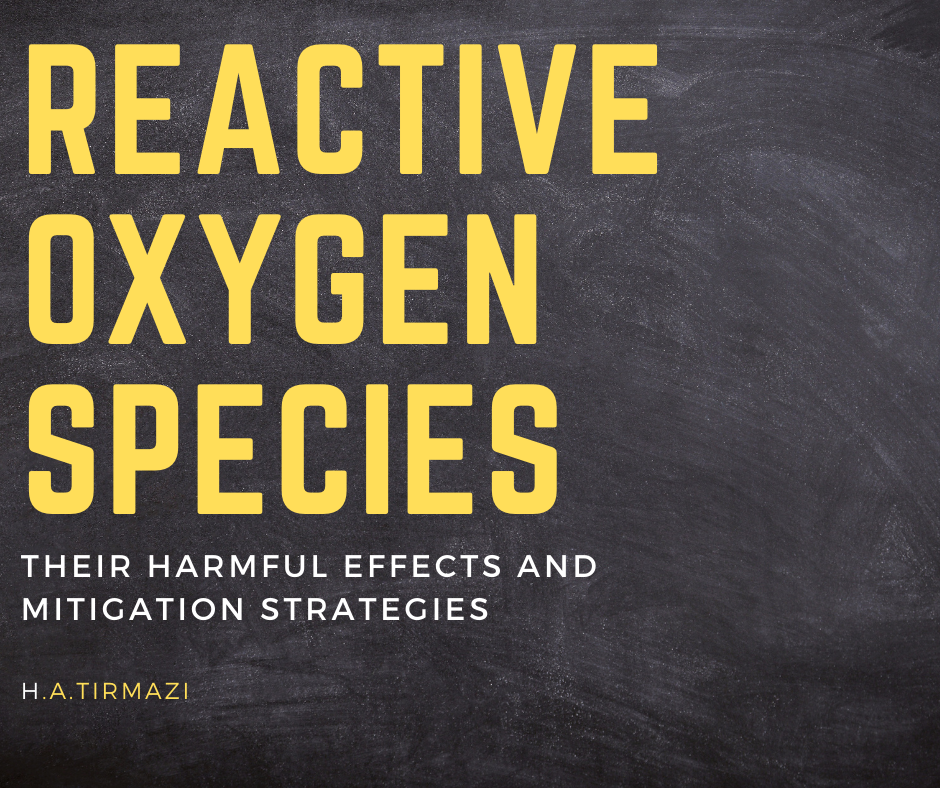
Reactive Oxygen Species (ROS):
By H.A.Tirmazi
Introduction:
Reactive Oxygen Species (ROS) is a phrase used to describe a number of reactive molecules and free radicals derived from molecular oxygen. This unstable configuration creates energy that is released upon reaction with adjacent molecules, such as proteins, lipids, carbohydrates, and nucleic acids. The majority of free radicals that damage biological systems are derived from oxygen and more generally referred to as “reactive oxygen species.”
Oxygen (O2) is an essential element for cell function and life. It plays an important role in a series of biochemical reactions occurring in the respiratory chain, which is responsible for most of the production of adenosine triphosphate (ATP), which provides the energy required for a multitude of cellular reactions and functions. Most reactive oxygen species are generated as by-products during mitochondrial electron transport
In addition ROS are formed as necessary intermediates of metal catalyzed oxidation reactions. Atomic oxygen has two unpaired electrons in separate orbits in its outer electron shell. This electron structure makes oxygen susceptible to radical formation. The sequential reduction of oxygen through the addition of electrons leads to the formation of a number of ROS including: superoxide; hydrogen peroxide; hydroxyl radical; hydroxyl ion; and nitric oxide
There are numerous cellular systems that can produce ROS. The major source of ROS production in the cell is the mitochondrial respiratory chain that utilizes approximately 80–90 % of the O2 a person consumes and generates most of the ROS produced in the body.
Another major source of ROS, especially in the liver, is a group of enzymes called the cytochrome P-450 mixed function oxidases. There are many variants of these iron-containing enzymes, some of which are responsible for removing or detoxifying a variety of compounds present in our environment and ingested (e.g., foods or drugs), including alcohol. Some cytochrome P-450 enzymes also are important for metabolizing substances that naturally occur in the body, such as fatty acids, cholesterol, steroids, or bile acids.
The cytochrome P-450 molecules in their biochemical reactions catalyzed use O2, and during these reactions small amounts of ROS are generated. The extent of ROS generated varies considerably depending on the compound to be degraded and on the cytochrome P-450 molecule involved. One type of cytochrome molecule that is especially active in producing ROS is known as CYP2E1, whose activity increases after heavy alcohol exposure. Other sources of ROS in the body are two types of immune cells called macrophages and neutrophils, which defend the body against invading microorganisms.
Harmful Effects Of ROS On Human:
Oxidative Stress:
ROS are highly reactive molecules. At low to moderate concentrations, they function in physiological cell processes, but can damage cell structures such as carbohydrates, nucleic acids, lipids, and proteins and alter their functions. The shift in the balance between oxidants and antioxidants in favor of oxidants is termed “oxidative stress.” Regulation of reducing and oxidizing (redox) state is critical for cell viability, activation, proliferation, and organ function.
ROS can lead to DNA modifications in several ways, which involves degradation of bases, single- or double stranded DNA breaks, purine, pyrimidine or sugar-bound modifications, mutations, deletions or translocations, and cross-linking with proteins. Most of these DNA modifications (Fig. 3) are highly relevant to carcinogenesis, aging, and neurodegenerative, cardiovascular, and autoimmune diseases. Tobacco smoke, redox metals, and non-redox metals, such as iron, cadmium, chrome, and arsenic, are also involved in carcinogenesis and aging by generating free radicals or binding with thiol groups.
Oxidative stress contributes towards many pathological conditions, including cancer, neurological disorders, atherosclerosis, hypertension, ischemia/perfusion, diabetes, acute respiratory distress syndrome, idiopathic pulmonary fibrosis, chronic obstructive pulmonary disease and asthma
Eosinophil peroxidase and MPO also contribute to the oxidative stress by modification of proteins by halogenations, nitration, and protein cross-links via tyrosyl radicals. Peroxidation of lipids disturbs the integrity of cell membranes and leads to rearrangement of membrane structure. Hyperoxia refers to conditions of higher oxygen levels than normal partial pressure of oxygen in the lungs or other body tissues. It leads to greater production of reactive oxygen and nitrogen species.
Cigarette smoke contains many oxidants and free radicals and organic compounds, such as superoxide and nitric oxide. In addition, inhalation of cigarette smoke into the lung also activates some endogenous mechanisms, such as accumulation of neutrophils and macrophages, which further increase the oxidant injury.
Heavy metal ions, such as iron, copper, cadmium, mercury, nickel, lead, and arsenic, can induce generation of reactive radicals and cause cellular damage via depletion of enzyme activities through lipid peroxidation and reaction with nuclear proteins and DNA. Arsenic is a highly toxic element that produces a variety of ROS, including superoxide (O2 -.), singlet oxygen (1O2), peroxyl radical (ROO_), nitric oxide (NO_), hydrogen peroxide (H2O2), and dimethylarsinic peroxyl radicals [(CH3)2AsOO_]. Arsenic (III) compounds can inhibit antioxidant enzymes, especially the GSH-dependent enzymes, such as glutathione-S transferases (GSTs), glutathione peroxidase (GSH-Px), and GSH reductase, via binding to their sulfhydryl (–SH) groups. Lead increases lipid peroxidation significant decreases in the activity of tissue SOD and brain GPx have been reported after lead exposure.
Mitigation Strategies for ROS:
Many people consume synthetic antioxidants in order to decrease oxidative stress, to modulate the aging process, and to extend the health-span. The key to the future success of decreasing oxidative-stress-induced damage should be the suppression of oxidative damage without disruption of the well-integrated antioxidant defense network. The approach to neutralize free radicals with antioxidants should be changed into prevention of free radical formation in the first place. Reducing the free radical formation should be more efficient than trying to neutralize free radicals after they have been produced. Among several mitigation and preventing strategies against harmful impacts of reactive oxygen species, some are discussed below:
1. Caloric Restriction:
The hormesis hypothesis of caloric restriction states that the underlying mechanism of dietary restriction is the activation of a defense response that evolved to help organisms to cope with the adverse conditions. Caloric restriction (CR) can also work as a hormesis agent. A growing body of evidence supports the hypothesis that CR, with no malnutrition and an adequate mineral intake, also works by decreasing oxidative stress. The theory unites previously disparate observations about ROS defenses, apoptosis, metabolic changes, stress resistance, and hormonal changes. Four pathways have been implicated in mediating the CR effect. These are the insulin-like growth factor (IGF-1)/insulin signaling pathway, the sirtuin pathway, the adenosine-monophosphate-(AMP-) activated protein kinase (AMPK) pathway, and the target of rapamycin (TOR) pathway.
A main causal factor pertaining to the reduction of oxidative stress in animals subjected to continued CR relates to a decrease in mitochondrial free radical generation. CR promotes a metabolic shift resulting in more efficient electron transport in the mitochondrial respiratory chain. It was reported that long-term CR led to a 45% decrease in the rate of mitochondrial H2O2 generation and 30% decrease in oxidative damage to mtDNA in the rat heart, and a 28% reduction in the rate of mitochondrial ROS generation and 30% decrease in oxidative damage to mtDNA in rat skeletal muscle. Mitohormesis or mitochondrial hormesis suggests that increased mitochondrial activity and specifically generation of oxidative stress due to this metabolic increase exert positive biological effects ultimately promoting health.
2. Mitochondrial Un-coupling:
Uncoupling is defined as a condition in which the rate of electron transport can no longer be regulated by an intact chemi-osmotic gradient. Uncoupling agents dissipate the chemical gradient, usually by creating a mechanism by which protons can escape the inter-membrane space (or otherwise “short out” the gradient), allowing an increase in electron transport. Mitochondrial uncoupling has been proposed as a mechanism that reduces reactive oxygen species production and could account for the paradox between longevity and activity. When oxygen consumption is uncoupled from ATP generation, heat is produced. However, the consumption of oxygen without ATP production would also reduce the level of free molecular oxygen potentially available for superoxide anion formation. Small reductions in metabolic flux through the electron transport chain occur at the cost of increased upstream substrate levels. This increased concentration of reduced upstream substrates allows a larger generation of ROS.
When the mitochondria are uncoupled and membrane potential is low, animals might actually produce less free radicals when expending the most energy. Examples of uncoupling agents are the uncoupling proteins (UPCs), a family of transporters belonging to the mitochondrial carrier protein super family, which is found in all eukaryotic organisms. An uncoupling protein is a mitochondrial inner membrane protein that can dissipate the proton gradient before it can be used to provide the energy for oxidative phosphorylation. Uncoupling proteins are present in mitochondrial inner membrane and mediate free fatty acid-activated, purine nucleotide- inhibited H+ reuptake. UCPs can modulate the tightness of coupling between mitochondrial respiration and ATP synthesis.
Uncoupling of oxidation of respiratory substrates and phosphorylation of ADP lowers the efficiency of ATP formation. At the same time, it may diminish the formation of ROS by decreasing the mitochondrial membrane potential and thereby stimulating the electron transport and oxygen consumption. This is due to shortening of the half-life of semiquinone, an intermediate of the electron transport system, which can transfer the electron to molecular oxygen, generating superoxide radicals, and then hydrogen peroxides.
3. Lifestyle Approaches for Oxidative Reduction:
Consumption of vegetables and plant-derived foods and beverages has positive effect on the prevention of age associated diseases like coronary heart disease and atherosclerosis as well as for longevity. Based on the analysis of available epidemiological data, a daily intake of at least 400 g of fruits and vegetables has been recommended by the World Health Organisation. Foods rich in antioxidants include all fresh and seasonal fruit and vegetables (peppers, apples, onions, pineapple, dark leafy vegetables, flaxseeds, walnuts, pumpkin seeds, and olives), olive oil, and fish. Also omega-3 fatty acids EPA and DHA appear to stabilize mitochondrial membranes as well as magnesium which is required for the oxidation reduction steps in mitochondria since magnesium deficiency enhances free radical formation.
Nutritional (or dietary) oxidative stress describes an imbalance between the prooxidant load and the antioxidant defense as a consequence of excess oxidative load or of an inadequate supply of the organism with nutrients. In Western societies, a significant part of the day is spent in the postprandial state. Postprandial (postprandial means after eating a meal, while pre-prandial is before a meal) oxidative stress is characterized by an increased susceptibility of the organism toward oxidative damage after consumption of a meal rich in lipids and/or carbohydrates. The “three meals a day plus snacks” diet is typical of many modern industrialized countries. In contrast to the continuous availability of food that we currently enjoy, our ancestors were often forced to endure extended periods from many hours to days without food. When overeating, high amounts of energy become available and mitochondria do not operate very efficiently and generate more superoxide. The electron transport always proceeds as fast as energy is removed from the gradient. Electron transport is driven by the free energy that is available from the energy carriers, in turn obtained from substrates such as glutamate or Krebs intermediates. It is restricted by the chemiosmotic gradient.
In all organisms, melatonin is produced primarily during the daily period of darkness, with only small amounts being synthesized during the day. For this reason, people should sleep long enough and in complete darkness in order to produce necessary amounts of the endogenous powerful antioxidant melatonin and benefit from its particular role in the protection of nuclear and mitochondrial DNA. Melatonin is an antioxidant that can easily cross cell membranes and the blood-brain barrier. Melatonin is a direct scavenger of OH, O2 −, and NO. Unlike other antioxidants, melatonin does not undergo redox cycling, the ability of a molecule to undergo reduction and oxidation repeatedly.
References:
· Poljsak, B., 2011. Strategies for reducing or preventing the generation of oxidative stress. Oxidative Medicine and Cellular Longevity, 2011.
· Popa-Wagner, A., Mitran, S., Sivanesan, S., Chang, E. and Buga, A.M., 2013. ROS and brain diseases: the good, the bad, and the ugly. Oxidative Medicine and Cellular Longevity, 2013.
· Kim, H., Yun, J. and Kwon, S.M., 2016. Therapeutic Strategies for Oxidative Stress-Related Cardiovascular Diseases: Removal of Excess Reactive Oxygen Species in Adult Stem Cells. Oxidative Medicine and Cellular Longevity, 2016.
· Bhattacharya, S., 2015. Reactive oxygen species and cellular defense system. In Free Radicals in Human Health and Disease (pp. 17-29). Springer India.
· Vatansever, F., de Melo, W.C., Avci, P., Vecchio, D., Sadasivam, M., Gupta, A., Chandran, R., Karimi, M., Parizotto, N.A., Yin, R. and Tegos, G.P., 2013. Antimicrobial strategies centered around reactive oxygen species–bactericidal antibiotics, photodynamic therapy, and beyond. FEMS microbiology reviews, 37(6), pp.955-989.
· Di Meo, S., Reed, T.T., Venditti, P. and Victor, V.M., 2016. Harmful and Beneficial Role of ROS. Oxidative Medicine and Cellular Longevity, 2016.
· Birben, E., Sahiner, U.M., Sackesen, C., Erzurum, S. and Kalayci, O., 2012. Oxidative stress and antioxidant defense. World Allergy Organization Journal, 5(1), p.9.
Comentários